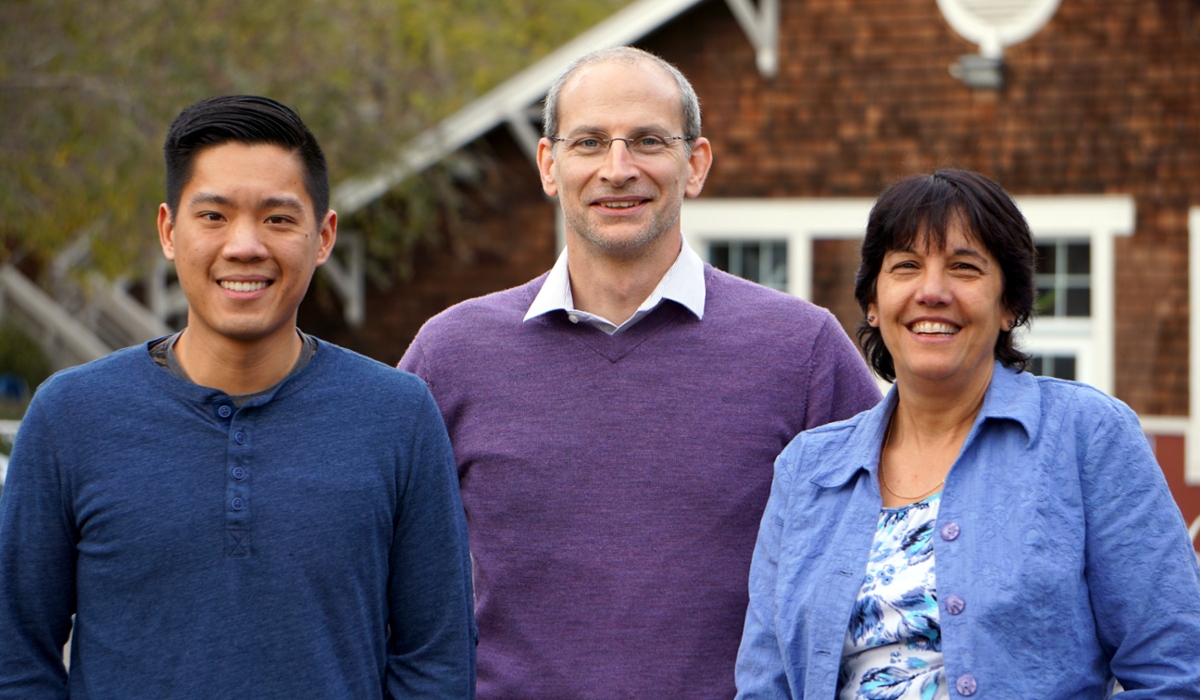
By Doug Banda
DNA damage repair enzymes are charged with the daunting task of guarding the cell's genetic blueprint from permanent changes, or mutations, which may arise from environmental toxins and internal cellular processes. If left unrepaired these mutations often lead to disease in humans, such as cancer, or to simple cell death in more primitive organisms such as bacteria and fungi. In order to survive, life has evolved an array of specialized repair enzymes known as DNA glycosylases to target damaged DNA nucleobases or their miscoding partners as substrates.
Until recently, little was known about the catalytic mechanism of DNA glycosylase AlkD, which repairs positively charged methylated bases, like 3-methyladenine (3mA), in duplex DNA. In 2008 and 2010 researchers in Professor Brandt Eichman's group at Vanderbilt University used substrate and product analogs to solve the first X-ray crystal structures of AlkD bound to duplex DNA (J. Mol. Bio. 2008, DOI: 10.1016/j.jmb.2008.05.078, Nature 2010, DOI: 10.1038/nature09428). However, these structures showed the methylated base positioned away from the enzyme in a shell of water, rather than being nestled within an enzyme active site. These structures suggested that either the enzyme worked by a completely unprecedented mechanism or that a non-productive complex had been trapped. It turns out both are true, and new work from researchers in the Chemistry Department at UC Davis in collaboration with the Eichman group challenges the dogma of a "base-flipping" mechanism by DNA glycosylases (Nature 2015, DOI: 10.1038/nature15728).
To further the story, Dr. Eichman wanted to produce new crystal structures that circumvent previous issues on the road to proposing a new AlkD mechanism. It was soon after that Dr. Eichman recruited Professor Sheila David and graduate student Phil Yuen to help elucidate the mechanism. "Eichman and I talked at a conference about the first structure and decided we could help them figure out what was happening," detailed Dr. David. The original crystal structures brought up a number of questions: Does AlkD go through base-flipping like canonical DNA glycosylases? What amino acid residues does it use to intercalate, or plug, into DNA? Does it use other strategies? To help answer some of these questions, Phil synthesized a DNA oligo sequence with a transition state analog, termed 1aR.
Like most enzymes, DNA glycosylases maximize the number of favorable interactions to the transition state of a given reaction in order to perform catalysis. So by utilizing the 1aR transition state analog, the Eichman group gained access to a new molecular tool to capture AlkD in a productive binding interaction with lesion-containing DNA. "By crystallizing 1aR with AlkD," explains Phil, "Dr. Elwood Mullins in the Eichman group was able to solve a set of crystal structures that provide a reaction scheme. Elwood was able to produce structures with the substrate analog (3d3mA), transition state/intermediate analog (1aR) and product analog (THF). These structures seemed to capture elements more in line with traditional DNA glycosylases that the first structures did not."
Together the two groups provided evidence to show that AlkD uniquely processes its substrate without a base-flipping mechanism or key amino acid residues that intercalate into the DNA duplex. When asked about the impact of the study, Dr. David tells that, "One of the coolest things about the structure in my opinion, is that it provides a means to cleave out bulky alkylated bases that would not be able to be housed in an enzyme active site. The Eichman groups' results showing the cleavage of yatakemycin-alkyated bases suggests that these are the types of substrates that AlkD processes naturally, not 3mA."
Though these guardians of the genome have been studied for years, new insights into their play in the chemical warfare of the cell continue to unfurl. Head over to the November 2015 issue of Nature to see the groups' full results and the story in its entirety.