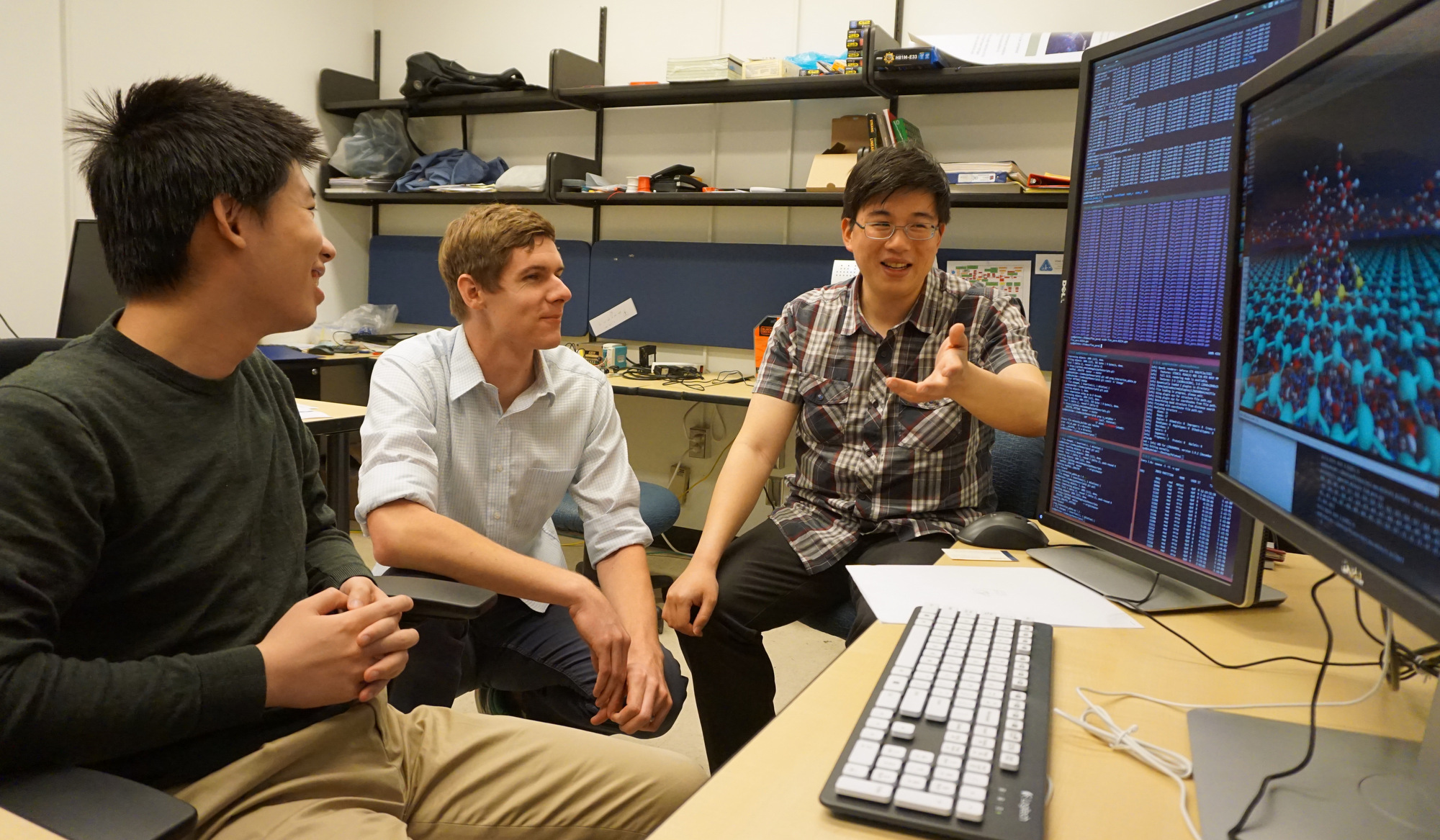
By Doug Banda
California is five years strong into the worst drought on state record. Those living in the Golden State aren't the only ones feeling parched, though--more than half-a-billion people around the world lack access to clean drinking water. The problem is so severe the United Nations predicts 1.8 billion people will be living in parts of the world with absolute water scarcity by 2025.
Many countries have turned to desalination plants as part of the solution, where ocean water is pumped at high pressures through semipermeable membranes to remove salts and other contaminants. This process, however, is costly and energy intensive: desalination plants consume over ten times the energy of traditional water treatment plants to produce the same volume of water. Researchers in the UC Davis Department of Chemistry are working together to investigate how to make this process less energy intensive and more efficient.
"I'm interested in any kind of chemical problem that has the potential for benefiting the environment," explains Dr. Lee-Ping Wang, echoing UC Davis' commitment to environmental and economic sustainability. He accepted a faculty position with the department in 2015. His research group develops and utilizes theoretical and computational chemistry methods to address issues in renewable and sustainable energy, like the water crisis currently affecting California and many other parts of the world. Dr. Wang and postdoctoral researcher Dr. Yudong Qiu are developing computational tools to study the use of nanoporous graphene membranes to improve reverse osmosis technology in desalination plants.
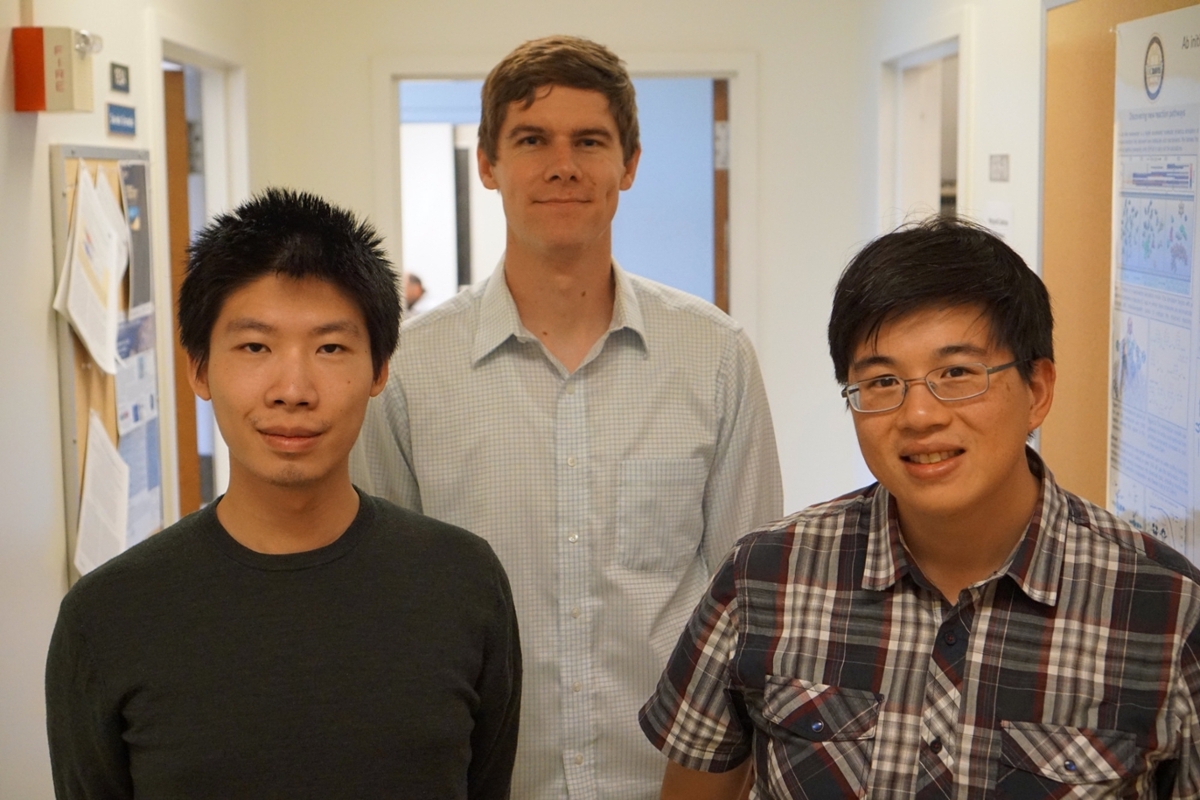
Graphene is a carbon nanomaterial consisting of a single layer of graphite. Researchers from Oak Ridge National Laboratory found in recent years that the rate of water purification is increased by 100,000-fold beyond existing theoretical predictions using nanoporous graphene. The experimentalists themselves have no explanation for the unexpectedly high performance and have proposed several hypotheses, such as the possibility that silicon atoms may be stabilizing the pore edges and speeding up water transport. Before this discovery can be used in desalination plants, researchers want to understand why the nanoporous membranes produce such a remarkable performance.
This is where Drs. Wang and Qiu step in, who possess the skill set needed to model and simulate this system through theoretical calculations. "We happen to have all the tools to give a high resolution atomic description of the interactions occurring when these membranes are used for water purification," explains Qiu. The two propose to do ab initio calculations (highly accurate quantum chemistry calculations that solve the electronic Schrödinger equation) to obtain information about the entire molecular system, including atomic charges, dipole moments and other energetic properties. This way one can describe systems like the interaction of a water molecule with a silicon atom accurately on a small scale.
With the information derived from ab initio calculations, Wang and Qiu will develop accurate force field descriptions of the interactions between atoms using an open-source software developed in the lab called ForceBalance. This will enable the simulation of water diffusion across these membranes on a larger scale. They propose to do this not only with graphene nanopores terminated with silyl hydrides (Si-H) but also with silanols (Si-OH) to further explore how silicon functionality affects the overall efficiency of water purification.
Looking forward, implementing this nanotechnology has the potential to bring fresh water to arid and coastal environments while reducing the energetic costs to do so.
The Wang Lab uses the power of computational chemistry to tackle diverse issues relating to the environment and renewable energy, which also include the mechanisms of electrochemical conversion of carbon dioxide to fuels. To learn more, head over to their webpage at http://www.lpwchem.org.